Mechanical Gasoline Fuel-Injection System with Lambda
Closed-Loop Control
Data from Bosch
|
Bosch
K-Jetronic
|
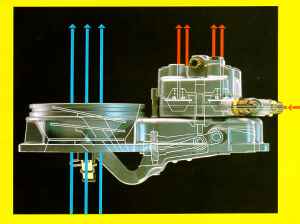 |
Part 2
|
Fuel Management
Mixture control unit
The task of fuel management is to meter, or allocate, the
correct quantity of fuel which corresponds to the amount of air
drawn in by the engine. Fuel management carried out by the mixture
control unit. This comprises the air-flow sensor and the fuel
distributor.
Air-flow sensor
The air-flow sensor operates according to the suspended-body
principle and measures the amount of air drawn in by the engine.
All the air drawn in by the engine flows through an air-flow
sensor which is connected upstream of the throttle plate. The
air-flow sensor is fitted with an air funnel in which is located a
movable sensor plate (the suspended body). The air drawn in
through the air funnel shifts the sensor plate by a certain amount
out of its zero position. The movement of the sensor plate is
transmitted to a control plunger by a lever system. This plunger
determines the quantity of fuel required. Considerable pressure
shocks can occur in the intake system if backfiring takes place in
the intake manifold. For this reason, the airflow sensor is so
designed that the sensor plate can swing back in the opposite
direction, past its zero position, and thus open a relief
cross-section in the funnel. A rubber buffer limits the swing-back
in the downward direction (in the case of the updraft air-flow
sensor, the swing-back in the upwards direction is also limited by
a rubber buffer). A leaf spring ensures that the sensor plate
assumes the correct zero position when the engine is stationary.
The sensor-plate movements are transmitted to the control plunger
in the fuel distributor by means of a lever system. The weight of
the sensor plate and the lever system are balanced by a
counterweight.
Fuel distributor
The fuel distributor meters (allocates) the correct amount of
fuel to the individual cylinders in accordance with the position
of the air-flow sensor plate. As already mentioned, the position
of the sensor plate is a measure of the amount of air drawn in by
the engine. The position of the plate is transmitted to the
control plunger by a lever. The control plunger controls the
amount of fuel which is to be injected. Depending upon its
position in the barrel with metering slits, the control plunger
opens or closes the slits to a greater or lesser degree. The fuel
flows through the open section of these slits to the differential
pressure valves and then to the fuel-injection valves. If
sensor-plate travel is only small, then the control plunger is
only lifted slightly and as a result only a small section of the
slot is opened for the passage of fuel. With larger plunger
travel, the plunger opens a larger section of the slits and more
fuel can flow. There is, therefore, a linear relationship between
sensor-plate travel and the slit section in the barrel, which is
opened for fuel flow. The force applied to the control plunger by
the sensor plate travel is opposed by another force, which comes
from the so-called control pressure. One of the functions of this
control pressure is to ensure that the control plunger follows the
movements of the sensor plate immediately and does not, for
instance, stay in the (upper) end position when the sensor plate
moves back down again. Further important functions of the control
pressure are discussed in the chapters dealing with warm-up and
full-load enrichment
Control pressure
The control pressure is tapped off from the primary pressure
through a restriction bore, which serves to decouple the
control-pressure circuit and the primary pressure circuit from one
another. A connection line joins the fuel distributor and the
warm-up regulator (control pressure regulator). When starting the
cold engine the control pressure is about 0.5 bar. As the engine
warms up, the warm-up regulator increases the control pressure to
about 3.7 bar. The control pressure acts through a damping
restriction on the control plunger and thereby develops the force,
which opposes the force of the air in the air-flow sensor. In
doing so, the restriction dampens a possible oscillation of the
sensor plate, which could result due to pulsating air-intake flow.
The control pressure influences the fuel distribution. If the
control pressure is low, the air drawn in by the engine can
deflect the sensor plate further. This results in the control
plunger opening the metering slits further and the engine being
allocated more fuel. On the other hand, if the control pressure is
high the air drawn in by the engine cannot deflect the sensor
plate so far and, as a result, the engine receives less fuel. In
order to fully seal off the control pressure circuit with absolute
certainty when the engine has been switched off, and at the same
time to maintain the pressure in the fuel circuit, the return line
of the warm-up regulator is fitted with a non-return valve. This
(push-up) valve is actually in the primary-pressure regulator and
is held open during operation by the pressure-regulator plunger.
When the engine is switched off and the plunger of the
primary-pressure regulator returns to its zero position, the
non-return valve is closed by a spring.
Differential-pressure valves
The differential-pressure valves in the fuel distributor serve
to hold the drop in pressure at the metering slits constant. The
air-flow sensor has a linear characteristic. This means that if
double the quantity of air is drawn in, the sensor-plate travel is
also doubled. If this (linear) travel is to result in a change of
delivered fuel in the same relationship, in this case double the
travel = double the quantity, then a constant drop in pressure
must be guaranteed at the metering slits independent of the amount
of fuel flowing through them. The differential-pressure valves
maintain the drop in pressure at the metering slits constant
independent of fuel through flow. The difference in pressure is
0.1 bar, this facilitates a high degree of control accuracy. The
differential-pressure valves are of the flat-seat type. They are
fitted in the fuel distributor and one such valve is allocated to
each metering slit. The upper and lower chambers of the valve are
separated by a diaphragm. The lower chambers of all the valves are
connected with one another by a ring main and are subjected to the
primary pressure (delivery pressure from fuel-supply pump). The
valve seat is located in the upper chamber. Each upper chamber is
connected to a metering slit and its corresponding fuel-injection
line. The upper chambers are completely sealed off from each
other. The diaphragms are spring-loaded and it is this helical
spring that produces the pressure differential. If more fuel flows
into the upper chamber through the metering slit, the diaphragm is
bent downwards and enlarges the valve cross-section at the outlet
line leading to the injection valve until the differential
pressure of 0.1 bar set by the spring again prevails. If less fuel
flows, the diaphragm bends back towards its original position and
decreases the valve cross-section at the outlet line until the
differential pressure of 0.1 bar is again present. This causes an
equilibrium of forces to prevail at the diaphragm which can be
maintained for every quantity of fuel by controlling the valve
cross-section.
Mixture formation
The formation of the air-fuel mixture takes place in the intake
manifold (tubes) and cylinders of the engine. The continually
injected fuel coming from the injection valves is
"stored" in front of the intake valves. When the intake
valve is opened, the air drawn in by the engine carries the
waiting "cloud" of fuel with it into the cylinder. An
ignitable air-fuel mixture is formed during the induction stroke
due to the swirl effect.
Mixture Adaptation
In addition to the basic functions described up to now, the
mixture has to be adapted during particular operating conditions.
These adaptations (corrections) are necessary in order to optimise
the power delivered, to improve the exhaust-gas composition and to
improve the starting behaviour and driveability
Cold start
Depending upon the engine temperature, the start valve injects
extra fuel into the intake manifold for a limited period during
the starting process. During cold starting, part of the fuel in
the mixture drawn in is lost due to condensation on the cold
cylinder walls. In order to compensate for this loss and to
facilitate starting the cold engine, extra fuel must be injected
at the instant of start-up. This extra fuel is injected by the
start valve into the intake manifold. The injection period of the
start valve is limited by a thermo-time switch depending upon the
engine temperature. This process is known as cold-start enrichment
and results in a "richer" air-fuel mixture, i.e. the
excess-air factor is temporarily less than 1.
Start valve
The start valve is of the solenoid-operated type. The winding
of an electromagnet is fitted inside the valve. In the inoperated
state, the movable armature of the electromagnet is forced against
a seal by means of a spring and thus closes the valve. When the
electromagnet is energised, the armature which as a result has
lifted from the valve seat opens the passage for the flow of fuel
through the valve. From here, the fuel enters a special nozzle at
a tangent and is caused to rotate. The fuel is particularly well
atomised by this specially shaped nozzle - the so-called
"swirl nozzle" - and enriches the air in the intake
manifold, downstream of the throttle valve, with fuel.
Thermo-time switch
The thermo-time switch limits the injection period of the start
valve dependent upon engine temperature. It is comprised of an
electrically heated bimetal strip which depending upon its
temperature either opens or closes an electric contact. The
complete device is fitted into a hollow threaded pin which in turn
is located at a position where typical engine temperature
prevails. The thermo-time switch determines the injection period
of the start valve. In doing so, the warming-up of the switch due
both to the engine heat and to the surrounding temperature, as
well as its in-built electrical heating filament are the
determining factors. The in-built heating facility is necessary in
order to limit the maximum start-valve injection period. The
mixture would otherwise become too rich and the engine would not
start due to "flooding". During cold start the injection
period depends mainly upon the electrical heating facility.
(Switch off at -20°C after approx. 8 seconds). On the other hand,
when the engine is already warmed-up the heat from the engine has
heated the thermo-time switch to such a degree that it remains
permanently open. As a result, an engine which is already at
operating temperature is not provided with extra fuel for
starting.
Warm-up
Warm-up enrichment is controlled by the warm-up regulator. When
the engine is cold the warm-up regulator reduces the control
pressure to a degree dependent upon engine temperature and thus
causes the metering slits to open further. At the beginning of the
warm-up period which directly follows the cold start, some of the
injected fuel still condenses on the cylinder walls and in the
intake ports. This can cause combustion miss to occur. For this
reason, the air-fuel mixture must be enriched during the warm-up
phase (Lambda<1.0). This enrichment must be continuously
reduced along with the rise in engine temperature in order to
prevent the mixture being over-rich when higher engine
temperatures have been reached. The warm-up regulator
(control-pressure regulator) is the component which carries out
this mixture control for the warm -up period by changing the
control pressure.
Warm-up regulator
The change of the control pressure is effected by the warm-up
regulator which is so fitted to the engine that it ultimately
adopts the engine temperature. In addition, the warm-up regulator
is electrically heated which enables it to be precisely matched to
the engine characteristic. It comprises a spring-controlled flat
seat diaphragm-type valve and an electrically heated bimetal
spring. In the cold state the bimetal spring exerts an opposing
force to that of the valve spring and, as a result, reduces the
effective pressure applied to the underside of the valve
diaphragm. This means that the valve outlet cross-section is
slightly increased at this point and more fuel is diverted out of
the control-pressure circuit in order to achieve a low control
pressure. As soon as the engine is cranked the bimetal spring is
heated electrically and after starting it is also heated by the
engine. The spring bends, and in doing so reduces the force
opposing the valve spring which, as a result, pushes up the
diaphragm of the flat-seat valve. The valve outlet cross section
is reduced and the pressure in the control-pressure circuit rises.
Warm-up enrichment is completed when the bimetal spring has lifted
fully from the valve spring. The control pressure is now solely
controlled by the valve spring and maintained at its normal level.
The control pressure is about 0.5 bar at cold start and about 3.7
bar with the engine at operating temperature.
Auxiliary-air device
In order to overcome the increased friction in the cold state
and to guarantee smooth idling, the engine receives more air-fuel
mixture during the warm-up phase due to the action of the
auxiliary-air device. When the engine is cold, the frictional
resistances are higher than when it is at operating temperature.
These must also be overcome by the engine during idle. For this
reason, the engine is allowed to draw in more air by means of the
auxiliary-air device which by-passes the throttle valve. Due to
the fact that this auxiliary air is measured by the air-flow
sensor and taken into account for fuel metering, the engine is
provided with more air-fuel mixture. This results in idle
stabilisation when the engine is cold. In the auxiliary-air device
a perforated plate is pivoted by means of a bimetal spring and
changes the open cross section of the bypass line. Dependent upon
temperature the plate assumes a given position, so that in the
case of a cold engine a correspondingly larger cross section of
the bypass line is opened. As the temperature increases the open
area is decreased until, finally, it is closed completely. The
bimetal is heated electrically. This means that the opening time
can be limited according to engine type. The auxiliary-air device
is so located that it is heated up by the engine to the engine
temperature. This ensures that the auxiliary-air device does not
respond when the engine is warm.
Load conditions
The adaptation, or correction, of the air-fuel mixture to the
operating conditions of idle, part load and full load is carried
out by means of appropriately shaping the air funnel in the
air-flow sensor. If the funnel had a purely conical shape, the
result would be a mixture with a constant air-fuel ratio
throughout the whole of the sensor plate range of travel (metering
range). As has already been mentioned though, it is necessary to
meter to the engine an air-fuel mixture which is optimal for
particular operating conditions such as idle, part load and full
load. In practice, this means a richer mixture at idle and full
load, and a leaner mixture in the part-load range. This adaptation
is achieved by designing the air funnel so that it becomes wider
in stages If the cone shape of the funnel is flatter than the
basic cone shape (which was specified for a particular mixture,
e.g. for Lambda= 1) this results in a leaner mixture. If the
funnel walls are steeper than in the basic model the sensor plate
is lifted further for the same air throughput, more fuel is
therefore metered and the mixture is richer. Hence, the funnel is
so shaped that a richer mixture is produced at idle and full load,
and a leaner mixture at part load (full-load and idle enrichment).
Mixture enrichment by means of control-pressure reduction In
those cases where engines are operated with a very lean mixture in
the part load range, an extra mixture enrichment must be provided
at full load in addition to the mixture adaptation resulting from
the shape of the air funnel. This extra enrichment is carried out
by a specially designed warm-up regulator. This regulates the
control pressure depending upon the manifold pressure. In this
model of the warm-up regulator, two valve springs are used instead
of one. The outer of the two springs is supported on the housing
as is the case with the normal-model warm-up regulator. The inner
spring though, is supported on a diaphragm which divides the
regulator into an upper and a lower chamber. The manifold pressure
is effective in the upper chamber which is connected to the intake
manifold, behind the throttle valve, by means of a hose. Depending
upon the model, the lower chamber is subjected to atmospheric
pressure either directly or by means of a second hose leading to
the air filter. Due to the low manifold pressure in the idle and
part-load ranges, which is also present in the upper chamber, the
diaphragm lifts to its upper stop. The inner spring is now at
maximum pretension. The pretension of both springs, as a result,
determines the particular control pressure for these two ranges.
When the throttle valve is opened further at full load, the
pressure in the intake manifold increases, the diaphragm leaves
the upper stops and is pressed against the lower stops. The inner
spring is relieved of tension and the control pressure reduced by
the specified amount as a result. In this manner, mixture
enrichment is achieved.
Acceleration response
The good acceleration response is a result of the sensor plate
"overswing". Acceleration During the transition from one
operating condition to the other, changes in the mixture ratio
occur which are utilised to improve the driveability. If at
constant engine speed the throttle valve is suddenly opened, the
amount of air which enters the combustion chamber, plus the amount
of air which is needed to bring the manifold pressure up to the
new level, flow through the airflow sensor. This causes the sensor
plate to briefly "overswing" past the fully opened
throttle point. This "overswing" results in more fuel
being metered to the engine (acceleration enrichment) and ensures
good acceleration response.
Controlling the air-fuel mixture
In order to adapt the injected fuel quantity to the ideal
air-fuel ratio of Lambda= 1, the pressure in the lower chambers of
the fuel distributor is varied. If for instance the pressure is
reduced, the differential pressure at the metering slots climbs
accordingly with the result that the injected fuel quantity is
also increased. In order to be able to vary the pressure in the
lower chambers, these are decoupled (in contrast to the
conventional K-Jetronic fuel distributor) from the primary
pressure. Decoupling is by means of a fixed throttle. A further
throttle connects the lower chambers with the fuel return. This
throttle is variable. If it is open, the pressure in the lower
chambers can reduce. If it is closed, the primary pressure is
present in the lower chambers. If this throttle is opened and
closed rapidly, it is possible to vary the pressure in the lower
chambers to correspond to the ratio between open time and close
time. An electromagnetic valve, the timing valve, is used as the
variable throttle. It is controlled by electrical pulses from the
Lambda control unit.
|